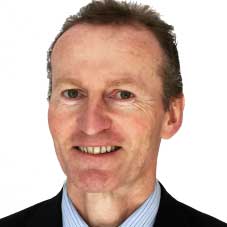
Technologies to image lesion creation in real time are required to improve the efficacy as well as the safety of atrial fibrillation (AF) ablation. By improving efficacy of ablation, the requirement and attendant risks of repeat atrial fibrillation ablation procedures may be obviated, writes David Keane (Dublin, Ireland). He reviews electrical indices, ultrasound, light, infrared, ultraviolet light with fluorescence and microwave technologies.
Efficacy
Recent studies have indicated a low rate of achieving persistent conduction block in patients in whom acute pulmonary vein isolation is demonstrated intra-procedurally.
In a recent multicentre study of ablation of persistent AF, Verma and colleagues found that acute procedural conduction block across left atrial linear lesions (LA roof and mitral isthmus lines) was achieved in only 74% of patients1. In a study of MRI findings post pulmonary vein (PV) isolation, Badger et al found complete circumferential scarring of all four PV antra was only achieved in 7% of patients2. In the GAP trial, recovery of conduction at three months to previously isolated pulmonary veins occurred in 70% of patients3, while in a study by Jiang et al, with follow-up at 12 months post pulmonary vein isolation, recovery of conduction to the PVs occurred in 90.6% of patients who had remained AF free post ablation4.
The above data indicate that demonstration of conduction block acutely is a poor differentiator between oedema with transient conduction block and permanent transmural myocardial necrosis.
Safety
Complications of AF ablation such as atrioesophageal fistula, vagal nerve injury, phrenic nerve injury, steam pop with cardiac perforation and endocardial disruption with stroke are felt to arise from excess lesion creation. The posterior left atrial wall has been found to have a mean myocardial thickness of only 1.7mm but a range of [1.1–2.6mm].5 Furthermore, the amount of periesophageal fat (thermal insulation and sink) varies considerably from patient to patient.
Technologies that can image atrial wall thickness and lesion creation in real time have the potential to avoid either excessive or inadequate lesion depth (the elusive Goldilock’s lesion).
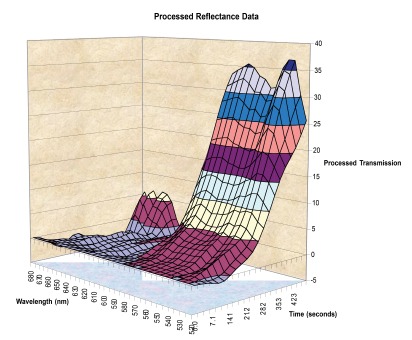
Technologies
Electrical indices
The rate of temperature rise upon radiofrequency (RF) application is an indicator of tissue contact while the rate of temperature decay upon RF offset is an indicator of lesion size (heat sink). Similarly, changes in impedance, electrogram amplitude reduction and pacing threshold, all correlate with lesion depth. Each parameter alone is of limited sensitivity and specificity for the determination of lesion transmurality6. The calculation of a combined multiparameter electrical index (electric index) will greatly increase the predictive value of electrical parameters.
This is analogous to formulae incorporating the force, power and time parameters of RF applications to predict lesion depth (eg. with a force of 15g, a power of 25W a transmural lesion on the posterior left atrial wall should be completed within 7s). Other parameters such as rate of irrigation, electrode endocardial interface sliding, jet ventilation (potential loss of sliding of the left atrium over the esophagus with more concentrated heat transfer at a single level of the esophagus) thickness of periesophageal fat (thermal insulation and sink) also potentially play a role in determining lesion depth.
Ultrasound-based technologies
Incorporation of the ICE transducer within the ablating electrode (rather than as a separate ICE catheter) guarantees that the area of interest is always in plane, the near field resolution is higher and the atrial lesion development, wall thickness and interface with the esophagus can be monitored in real time during ablation.7 Furthermore, incorporation of the ICE transducer obviates the risks attendant with a separate stand-alone catheter (mechanical cardiac and vascular injury).
An ICE platform may also be utilised to provide acoustic radiation force impulse imaging (ARFI)8 and photoacoustic imaging (PA).9 These techniques monitor the progressive loss of elasticity of myocardium during ablation. Healthy myocardium is compliant and is displaced by a sound wave while ablated myocardium through denaturation of proteins becomes stiffer. While both techniques image the response by ICE, ARFI utilises sound waves to displace the myocardium while PA utilises laser pulses to induce thermoelastic expansion and acoustic transients providing an optical absorption map for coregistration with ICE images.
Light-based technologies
Visible spectrum light imaging of the endocardium (endoscopy – with an optical fiber and translucent laser balloon) provides interesting real-time guidance to contact and energy delivery. However, limiting imaging to the composite visible spectrum and the reliance on the perception of the operator does not take advantage of the full potential of light.
As the myocardium is ablated the overall reflectance of light changes but this is more marked for certain wavelengths compared to others. Specifically, the proportion of light reflected in the green bandwidth increases more than the red bandwidth.10 Digital analysis of this pattern (reflectance spectroscopy) has the potential to provide real-time feedback on lesion formation (Figure 1).
Infrared technologies
Infrared transblood imaging using a wavelength sufficiently long to image through red blood cells (per Mie’s Theorem) can provide high resolution near field imaging of the electrode endocardial interface and offers significant potential value particularly if coupled with a translucent electrode.
Optical coherence tomography (OCT) provides high resolution imaging to 3mm depth of myocardium.11,12 OCT uses 1,300nm wavelength light to provide back reflected tomographic gray-scale imaging with a resolution of 10 microns. Changes in the myocardial architecture and birefringence of the highly ordered collagen and actin myosin complexes are visualised in real time during ablation (Figure 2). This technique is particularly useful at detecting subendocardial changes, which occur prior to steam pop formation and endocardial disruption and subsequent thrombus formation and thus potentially reduce the risk of cardiac perforation and stroke.
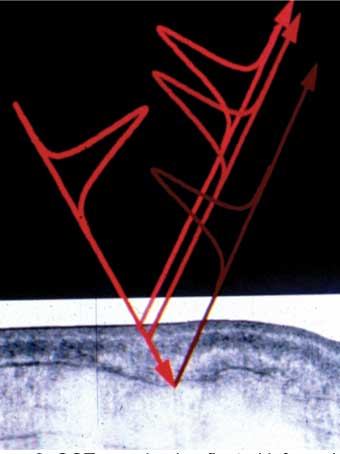
Ultraviolet light with fluorescence
When exposed to ultraviolet light, the myocardium produces a characteristic fluorescence response, due to the relatively high concentration of nicotinamide adenine dinucleotide (NADH). Healthy tissue emits strongly between 450nm and 470nm when excited between 340nm and 360nm, due to the presence of NADH. Ablation results in a continuous reduction in magnitude of the NADH fluorescence response as more tissue dies. This technique clearly separates oedema from ablated tissue and also provides indirect information on catheter contact pressure.
Microwave
Microwave radiometry measures electromagnetic energy considered as thermal radiation. This passive sensing microwave radiometry technology detects the temperature of myocardium beneath the endocardium. With this technology the rate of increase of temperature (>1.5 ◦C/s) has been found to be the single most powerful predictor of pop formation.13 It overcomes the limitation of contact thermocouples and thermistors, which are limited to surface temperature measurement underestimating the temperature of the underlying mid myocardium. Irrigated RF ablation drives the point of maximum temperature from the subendocardium to a deeper level (indeed for the thin posterior left atrial wall care needs to be given to avoid excessive deep tissue heating in the esophagus with relative sparing of the endocardium). Given that most RF ablation catheters used for AF ablation are now irrigated, microwave radiometry holds promise as a more reliable indicator of mid-myocardial tissue temperature.
Future directions
Parameters that are also being targeted for future developments include assessment of the degree of cardiac compression, stretch and compliance (Figure 3) as well as improved real-time transmural imaging of the anterior esophageal wall.
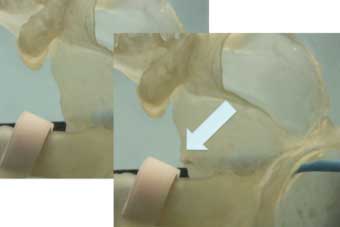
Non-invasive MRI thermography shows promise for determination of volumetric heating but may be most useful if coupled with technologies that provide non-invasive cardio-respiratory gated ablation.
Conclusion
Much of the real estate at the tip of an ablating catheter is already used up by contact force measurement, irrigation, temperature measurement, in addition to the primary electrode functions. The reimbursement rate for procedures by Medicare and Medicaid is unlikely to rise and thus there may be greater interest in technologies that reduce the cost of ablation procedures rather than the adoption of more sophisticated and potentially more expensive technologies. That said, if a technology can be shown to reduce the risk of complications it will be a most welcome development.
The recent findings that routine performance of a TEE pre PVI may increase esophageal injury14 and that the use of esophageal temperature monitor probes may increase esophageal lesions15 make it important that improvement in safety is demonstrated prior to routine adoption of new strategies.
References
- Atul Verma et al on behalf of the STAR AF 2 investigators – ESC 2014
- T Badger et al, Circ Arrhythm Electrophysiol 2010;3:249-259
- Kuck KH et al, GAP AF trial – Late-breaking clinical trials, ESC 2013. European Heart Journal 2014;35,1163–1164
- Jiang R et al, HeartRhythm 2014;11:969-976
- Hassink R et al, J Am Coll Cardiol 2003;42:1108–14
- Keane D et al, PACE 2009; 32:1543–1552.
- Wright M et al, HeartRhythm 2011;8:304 –312
- Eyerly S, Hsu S, Agashe S, Trahey G, Li Y, Wolf P. An In Vitro Assessment of Acoustic Radiation Force Impulse Imaging for Visualizing Cardiac Radiofrequency Ablation Lesions. J Cardiovasc Electrophysiol 2010;21:557-563
- Dana N, DiBiase L, Natale A, Emelianov S, Bouchard R. In vitro photoacoustic visualization of myocardial ablation lesions. HeartRhythm 2014;11:150–157
- Doshi S, Keane D. Catheter Microwave, Laser, and Ultrasound: Biophysics and Applications. In: Huang SKS, Wood MA, eds. Catheter Ablation of Cardiac Arrhythmias. Elsevier Saunders. ISBN-13: 978-1-4160-0312-0. 2006; 69-82
- Keane D et al, J Am Coll Cardiol 887-2; 163A
- Fleming C et al, Journal of Innovations in Cardiac Rhythm Management 2011;2:199–201
- Koruth J et al, J Cardiovasc Electrophysiol 2013;24:1271–1277
- Kumar S et al, J Cardiovasc Electrophysiol 2014 Oct 28. doi: 10.1111/jce.12575
- P Müller, JW Dietrich, P Halbfass, A Abouarab, F Fochler, A Szöllösi, K Nentwich, M Roos, J Krug, A Schade, A Mügge, T Deneke. Higher incidence of esophageal lesions after AF ablation related to the use of esophageal temperature probes. HeartRhythm 2015:in press
David Keane is director Cardiac Arrhythmia Service, St Vincent’s University Hospital, Dublin, Ireland