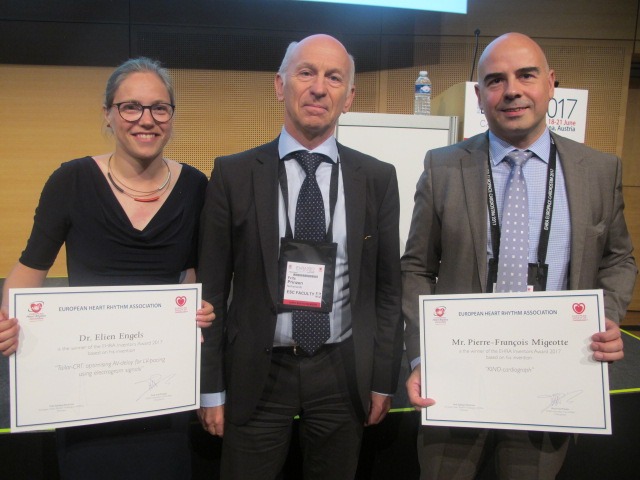
For the second-year running, the European Heart Rhythm Association (EHRA) held the EHRA Inventors Award competition at EHRA-EUROPACE CARDIOSTIM (18‒21 June, Vienna, Austria), a key activity part of the EHRA Innovation Forum which seeks to facilitate innovation in the cardiac rhythm field. Out of seven innovative projects, three were selected for presentation at the scientific meeting, which focused on cardiac remote monitoring and cardiac resynchronisation therapy (CRT).
The first and second best presentations were awarded with €3,000 and €2,000, respectively, which will either be used “to help further develop the innovation or to help the inventor go on a course to become a better inventor,” said EHRA Innovation Committee chair and chairman of the session, Frits Prinzen (Cardiovascular Research Institute Maastricht, Maastricht, the Netherlands). “The criteria to select the winners involved the effect of the invention to impact clinical practice and how far is the development supported by evidence,” added Prinzen.
Cardiac Rhythm News speaks to winner, Pierre–François Migeotte (Erasmus Hospital, Brussels, Belgium), who presented the Kino-Cardiograph, an automated non-invasive tool that can quickly provide feedback on all aspects of the cardiac function with self-monitoring at home, initially designed for atrial fibrillation and heart failure patients; and runner-up Elien B Engels (Maastricht University, Maastricht, the Netherlands), presenter of a patient-specific algorithm using electrograms from the pacemaker leads for cardiac resynchronisation therapy (CRT) optimisation.
Jonathan Behar (Barts Heart Centre, London, UK), third finalist of the EHRA Inventors Award, has also been featured in this article. He describes the details of the Guide CRT platform, a novel imaging technology designed to facilitate optimal left ventricular (LV) lead positioning in CRT.
Pierre–François Migeotte and the Kino-Cardiograph for cardiac remote monitoring
What is the rationale to develop the Kino-Cardiograph?
The Kino-Cardiograph was developed for the remote monitoring of the overall (electrical and mechanical) function of the heart in astronauts staying in the international space station for up to six months. Like a patient at home, the astronauts are up there far away from a medical doctor or a cardiologist. Thus a simple automated non-invasive tool that can quickly provide feedback on all aspects of the cardiac function was needed. We also identified that there is a gap in the cardiac monitoring at the hospital discharge when the patient is moving home. So the objective was to propose a system that could prevent rehospitalisation with an early identification of worsening condition and patient engagement with self-monitoring at home.
How does the technology work?
The Kino-Cardiograph is made of two connected small wearable patches—one close to the heart (apex) and the other placed in the lumbar region. They collect a traditional electrocardiogram (ECG) together with body vibrations recorded via miniature accelerometers. A one-minute recording at-rest provides enough data. A smartphone is used to pilot the device and transfer the data in the cloud on the centres’ server where they can be consulted by an expert and where artificial intelligence is built to interpret acceleration data, which provide the information on the mechanical function of the heart in terms of cardiac force and heart kinetic energy; hence the name Kino-Cardiograph for the device and Heartkinetics for the name of the Spin-off project from the Cardiology Department of the Erasmus Hospital and Université libre de Bruxelles, to clearly refer to the heart kinetic energy.
Which patients will potentially benefit?
The Kino-Cardiograph could benefit any patient that requires cardiac home-monitoring where more specific data than weight or blood pressure is needed. Atrial fibrillation and heart failure patients are targeted for first application. Indeed, currently there are no solutions for assessing the mechanical function of the heart at home.
What is the main clinical application?
The Kino-Cardiograph could also be used in hospital as an easy-to-use and efficient patient monitoring outside the intensive care unit where lighter solutions might benefit a larger number of patients. It could also potentially be used as a quick tool for diagnosis of atrial fibrillation or heart failure.
What are the key results of your clinical validation research?
The technology was tested in 34 healthy subjects in a randomised double-blind placebo vs. dobutamine trial where three different concentrations were infused in increasing steps. It was demonstrated that the Kino-Cardiograph could correctly sort the recordings from the highest dose to the lowest with intra-subject sensitivity of 96.91% and specificity of 98.01%. We developed machine learning algorithms to use a technique to detect (in inter-subject settings) increased contractility with high 90.3% negative predictive value (NPV) and 80.8% positive predictive value (PPV). In a 60 days bed-rest de-conditioning trial performed on 12 healthy subjects, our machine learning reached 93.1% NPV and 84.2% PPV to blindly detect the end-trial decrease in cardiac function. These numbers are very encouraging but need further validation.
What are the next steps of development?
The next steps are to further develop the clinical evidences in our capacity of monitoring heart-failure patients and to start the regulatory process for medical certification. We also aim to build an early-adopters user or beta-tester base to validate our concepts and key features and are encouraging healthcare professionals to contact us. Eventually, in about a year, we aim to incorporate the Heartkinetics company while starting sponsored clinical trials.
Elien B Engels and the 2D EGMV algorithm for CRT optimisation
What is the rationale to develop the 2D EGMV algorithm?
CRT is an established therapy for patients with heart failure and ventricular dyssynchrony, mainly due to left bundle branch block (LBBB). Large randomised trials have shown that CRT improves both morbidity and mortality. However, there is a considerable individual variability in CRT response. One of the reasons why patients do not respond to CRT is suboptimal atrioventricular (AV; delay between atrium and ventricle) timing. Since the AV-delay impacts the amount of fusion of intrinsic conduction with the paced activation wave, timing of the AV-delay during left ventricular (LV)-only pacing plays a crucial role. Preferably, the optimal AV-delay can be found automatically and continuously. The two-dimensional (2D) electrogram (EGM)-based vectorloop (EGMV) algorithm is patient-specific and can be embedded in the CRT device, such that it can perform automatic, continuous and ambulatory optimisation of the AV-delay using electrogram signals from unpaced pacing electrodes.
How does the technique work?
During CRT, the unipolar EGM signals of the unpaced pacemaker electrodes are recorded. From these unipolar EGM signals, two bipolar EGMs can be calculated by subtracting the right ventricular (RV) ring unipolar EGM signal from the distal LV electrode or from the proximal LV electrode. Plotting these bipolar EGM signals against each other forms a 2D EGM vector (EGMV). The longest AV-delay at which the maximal amplitude of the QRS complex is equal to the amplitude during pacing at a very short AV-delay is equal to the optimal AV-delay.
Which patients will potentially benefit?
All patients receiving CRT. For the CRT responders, it might increase the benefit of CRT even further, while the non-responders might become CRT responders due to AV-delay optimisation.
What is the main clinical application?
Optimisation of the AV-delay during CRT.
What are the key results of your clinical validation research?
The algorithm was tested in 25 CRT patients. It was shown that the EGMV can be used to find the optimal AV-delay to perform LV fusion pacing. It can do so by optimising individually—taking the actual resynchronisation into account. The algorithm also showed the ability to perform continuous optimisation as well as in an ambulatory fashion. (The acute validation of this algorithm has been published by Engel EB et al, Europace 2017; 0:1‒8 https://doi.org/10.1093/europace/eux208)
What are the next steps of development?
Develop and test software to automatically sense changes in QRS amplitude and hereby automatically adjusts the AV-delay. A non-inferiority clinical study comparing echocardiographic and clinical benefit of the 2D EGMV technique and AdaptivCRT (Medtronic) algorithm would be a next step.
The patent of the 2D EGMV algorithm was filed in 2013 (#9,248,294 FW Prinzen, EB Engels, A Aranda) and 2017 in collaboration with Medtronic.
Cardiac resynchronisation therapy guided by imaging: The next step for optimal left ventricular lead positioning
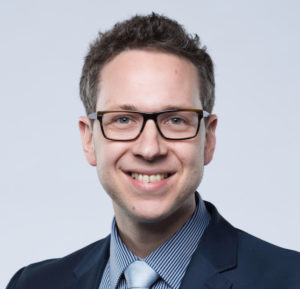
Jonathan Behar (London, UK) co-inventor for the Guide CRT platform—shortlisted as a 2017 finalist for the EHRA Inventors Award—details the benefits of this novel technology, which enables the real-time analysis and fusion of cardiac magnetic resonance imaging (MRI)-derived scar and dyssynchrony data to guide LV lead implantation in cardiac resynchronisation therapy.
Cardiomyopathies can cause electrical disturbance and slow myocardial conduction, resulting in a delay between left and right ventricular activation, most often seen as LBBB.1 A subsequent disco-ordinated, inefficient biventricular contraction results in wasted energy, impaired cardiac output2 and progressive left ventricular dilatation3 leading to significant morbidity and mortality. Biventricular pacing or cardiac resynchronisation therapy (CRT) is a highly effective electrical therapy for selected heart failure patients with persistent symptoms despite optimal medical therapy, prolonged QRS duration and a severely depressed left ventricular (LV) systolic function. It is thought to act by restoring electro-mechanical synchrony.4
Suboptimal response to CRT
Despite delivering CRT for over two decades, a significant proportion (30‒50%) fail to improve as demonstrated in the 2012 EHRA survey of CRT.5 Disappointingly, this proportion has remained static over a period of extensive technology and technique development. Causes of suboptimal CRT response are multi-factorial and include suboptimal LV lead placement, ineffective electro-mechanical resynchronisation, untreated anaemia and ineffectual atrioventricular (AV) and ventriculoventricular (VV) timings with respect to device optimisation.6
The evidence-base for image guidance
An increasing number of clinical studies have demonstrated the importance of avoiding areas of myocardial fibrosis and targeting regions of latest mechanical activation or greatest dyssynchrony, as an approach to identifying a target for LV lead placement. TARGET and STARTER were both single-centre randomised controlled studies evaluating the use of speckle tracking echocardiography for pre procedural targeting of myocardial segments for LV lead delivery, as compared with conventional non-image guided implantation.7,8 Patients in whom an LV lead was placed in a region of latest mechanical activation had significantly reduced rates of heart failure hospitalisation and death compared with those where the lead was distant. A large retrospective registry of 559 patients in whom pre procedural cardiac MRI was performed, demonstrated that patients with the LV lead implanted in areas of myocardial fibrosis had a greater hazard ratio of cardiovascular death or heart failure hospitalisation compared with those where the lead was out of scar (HR 5.57, p<0.0001). More recently, a selection of multi-modality imaging studies have shown the additional benefit for avoiding scar and targeting dyssynchrony amongst patients being implanted with CRT.9‒11 A common theme amongst these studies is the observed improvement in CRT response rate (approximately 15‒20%) in those implanted using an image-guided approach.
Real-time image fusion to guide CRT
Whilst the results from the growing number of image guidance studies is encouraging, one of the major limitations is that the data output from the imaging modality in each study is reviewed separate from, and alongside, X-ray rather than integrated together. Given the radiolucency of the cardiac silhouette and high variability in the rotation of the left and right-sided chambers relative to one another, it is not altogether surprising that using fluoroscopy to determine regional anatomy can be highly inaccurate, particularly with regard to CRT and lead position.12
Earlier this year, our group published the first clinical study to evaluate a platform (Guide CRT) which enables the real-time analysis and fusion of cardiac MRI-derived scar and dyssynchrony data to guide LV lead implantation.13 Upon completion of a routine cardiac MRI scar, the patient is transferred to the adjacent cardiac catheter lab (X-MRI suite) whilst simultaneously, the imaging dataset is processed within 25 minutes. Segmentation of long and short axis MRI sequences generates a 3-dimensional (3D) mesh and the Guide CRT display is shown detailing the location, burden and transmurality of myocardial fibrosis, in addition to volume vs. time curves for the 16-American Heart Association (AHA) segments, in order to identify regions of greatest dyssynchrony (Figure 1).
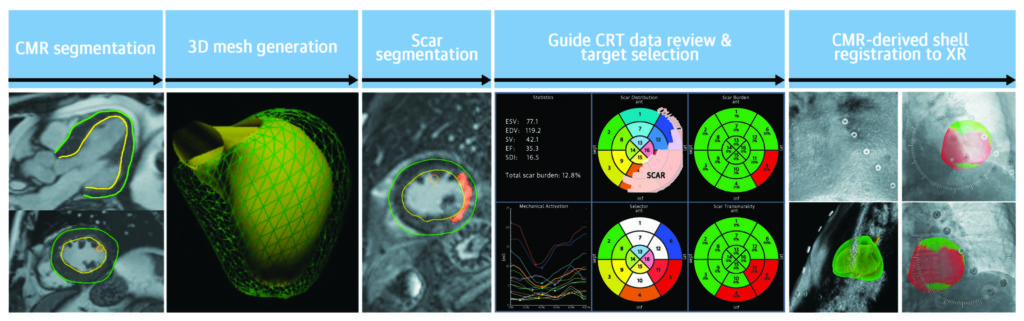
Following a short registration step, the 3D model is registered to the X-ray co-ordinate system and crucially, any subsequent X-ray acquisitions during the case are displayed with instantaneous overlay of the correctly oriented 3D model. Upon coronary venography, the 3D derived model of the patient’s left ventricle is instantaneously fused enabling the implanting physician to identify the patient specific target locations for LV lead placement and how these are subtended by the coronary venous tree (Figure 2).
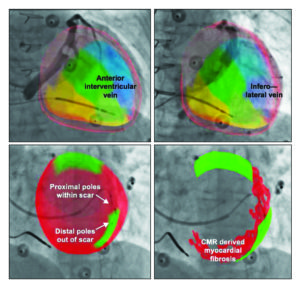
This platform was safely tested in 14 patients with conventional indications for CRT. It demonstrated that pacing with cardiac MRI-derived segments out of scar had more favourable electrical properties (lower capture thresholds – mean difference 2.4 Volts (1.5-3.2), p<0.001 and lower paced QRS duration – mean difference 25ms (15‒34), p<0.001) as compared with pacing within cardiac MRI-derived regions of scar). The mixed effect model analysis validated the accuracy of the cardiac MRI-derived, fused 3D model.
Personalised therapy for the 21st century
Empirical placement of the LV lead in the inferolateral wall has been the standard of care for the past two decades of CRT implantation and yet there are clear data to show the optimal LV site is highly patient specific.14 Utilising the individual’s coronary venous anatomy, scar distribution, electrophysiology and mechanical contraction patterns to identify and target the optimal site for the LV lead is one novel approach toward improving the effectiveness of CRT. Larger scale, multicentre, randomised clinical trials will be required to demonstrate whether this approach yields greater CRT response compared with the conventional implantation method.
The recent emergence of LV endocardial stimulation as an alternative route to delivering biventricular pacing for CRT has a growing evidence base15 with some data showing superior haemodynamic and electrophysiological indicies16‒19. The use of the image guidance strategies described may be particularly suitable for this approach with the ability to truly target any region on the LV endocardial wall without the constraint of the coronary venous anatomy. Recent development of a wireless intracardiac LV endocardial electrode for CRT delivery (WiSE-CRT, EBR systems) represents a unique opportunity to use integrated multi modality image guidance for optimal LV site selection (Figure 3).20
References:
- Grines CL, Bashore TM, Boudoulas H, Olson S, Shafer P, Wooley CF. Functional abnormalities in isolated left bundle branch block. The effect of interventricular asynchrony. Circulation [Internet]. American Heart Association Journals; 1989 Apr [cited 2016 Jul 11];79(4):845–53. Available from: http://www.ncbi.nlm.nih.gov/pubmed/2924415
- Prinzen FW, Augustijn CH, Arts T, Allessie MA, Reneman RS. Redistribution of myocardial fiber strain and blood flow by asynchronous activation. Am J Physiol [Internet]. 1990 Aug [cited 2016 Jul 10];259(2 Pt 2):H300-8. Available from: http://www.ncbi.nlm.nih.gov/pubmed/2386214
- Abraham WT, Hayes DL. Cardiac resynchronization therapy for heart failure. Circulation [Internet]. American Heart Association Journals; 2003 Nov 25 [cited 2016 Jul 10];108(21):2596–603. Available from: http://www.ncbi.nlm.nih.gov/pubmed/14638522
- Cazeau S, Ritter P, Bakdach S, Lazarus A, Limousin M, Henao L, et al. Four chamber pacing in dilated cardiomyopathy. Pacing Clin Electrophysiol [Internet]. Blackwell Publishing Ltd; 1994 Nov [cited 2016 Jul 10];17(11):1974–9. Available from: http://doi.wiley.com/10.1111/j.1540-8159.1994.tb03783.x
- Daubert J-C, Saxon L, Adamson PB, Auricchio A, Berger RD, Beshai JF, et al. 2012 EHRA/HRS expert consensus statement on cardiac resynchronization therapy in heart failure: implant and follow-up recommendations and management. Europace [Internet]. 2012 Sep [cited 2015 Jan 7];14(9):1236–86. Available from: http://www.ncbi.nlm.nih.gov/pubmed/22930717
- Mullens W, Grimm R a, Verga T, Dresing T, Starling RC, Wilkoff BL, et al. Insights from a cardiac resynchronization optimization clinic as part of a heart failure disease management program. J Am Coll Cardiol [Internet]. American College of Cardiology Foundation; 2009 Mar 3 [cited 2014 Nov 16];53(9):765–73. Available from: http://www.ncbi.nlm.nih.gov/pubmed/19245967
- Khan FZ, Virdee MS, Palmer CR, Pugh PJ, O’Halloran D, Elsik M, et al. Targeted left ventricular lead placement to guide cardiac resynchronization therapy: the TARGET study: a randomized, controlled trial. J Am Coll Cardiol [Internet]. Elsevier Inc.; 2012 Apr 24 [cited 2014 Nov 12];59(17):1509–18. Available from: http://www.ncbi.nlm.nih.gov/pubmed/22405632
- Saba S, Marek J, Schwartzman D, Jain S, Adelstein E, White P, et al. Echocardiography-guided left ventricular lead placement for cardiac resynchronization therapy: results of the Speckle tracking assisted resynchronization therapy for electrode region trial. Circ Heart Fail [Internet]. 2013 May [cited 2014 Nov 15];6(3):427–34. Available from: http://www.ncbi.nlm.nih.gov/pubmed/23476053
- Bertini M, Mele D, Malagù M, Fiorencis A, Toselli T, Casadei F, et al. Cardiac resynchronization therapy guided by multimodality cardiac imaging. Eur J Heart Fail [Internet]. John Wiley & Sons, Ltd; 2016 Nov [cited 2016 Nov 6];18(11):1375–82. Available from: http://doi.wiley.com/10.1002/ejhf.605
- Sommer A, Kronborg MB, Nørgaard BL, Poulsen SH, Bouchelouche K, Böttcher M, et al. Multimodality imaging-guided left ventricular lead placement in cardiac resynchronization therapy: A randomized controlled trial. European Journal of Heart Failure. 2016; 18(11):1365‒1374
- Nguyên UC, Mafi-Rad M, Aben J-P, Smulders MW, Engels EB, van Stipdonk AMW, et al. A novel approach for left ventricular lead placement in cardiac resynchronization therapy: Intra-procedural integration of coronary venous electro-anatomic mapping with delayed enhancement cardiac magnetic resonance imaging. Heart Rhythm [Internet]. 2016 Sep 20 [cited 2016 Oct 5]; Available from: http://www.ncbi.nlm.nih.gov/pubmed/27663606
- Sommer A, Kronborg MB, Nørgaard BL, Gerdes C, Mortensen PT, Nielsen JC. Left and right ventricular lead positions are imprecisely determined by fluoroscopy in cardiac resynchronization therapy: a comparison with cardiac computed tomography. Europace [Internet]. 2014 Sep [cited 2014 Nov 16];16(9):1334–41. Available from: http://www.ncbi.nlm.nih.gov/pubmed/24687965
- Behar JM, Mountney P, Toth D, Reiml S, Panayiotou M, Brost A, et al. Real-Time X-MRI-guided left ventricular lead implantation for targeted delivery of cardiac resynchronization therapy. JACC Clin Electrophysiol [Internet]. 2017 Apr [cited 2017 Jul 9]; Available from: http://www.sciencedirect.com/science/article/pii/S2405500X17301305
- Derval N, Steendijk P, Gula LJ, Deplagne A, Laborderie J, Sacher F, et al. Optimizing hemodynamics in heart failure patients by systematic screening of left ventricular pacing sites: the lateral left ventricular wall and the coronary sinus are rarely the best sites. J Am Coll Cardiol [Internet]. Elsevier Inc.; 2010 Feb 9 [cited 2014 Nov 14];55(6):566–75. Available from: http://www.ncbi.nlm.nih.gov/pubmed/19931364
- Morgan JM, Biffi M, Gellér L, Leclercq C, Ruffa F, Tung S, et al. Alternate site cardiac resynchronization (ALSYNC): a prospective and multicentre study of left ventricular endocardial pacing for cardiac resynchronization therapy. Eur Heart J [Internet]. 2016;ehv723. Available from: http://eurheartj.oxfordjournals.org/lookup/doi/10.1093/eurheartj/ehv723
Jonathan Behar is a cardiology registrar at Barts Heart Centre, London, UK. He is a registered co-inventor for the Guide CRT platform described above. The Guide CRT platform has been submitted to obtain European patent
CARDIOSTIM Innovation Award 2017
Alongside the EHRA Inventors Award, CARDIOSTIM held the Innovation Award 2017. This award honours performances, achievements and cutting-edge technologies of manufacturers that are created to modernise professionals practice and improve patients’ care. The CARDIOSTIM Committee, chaired by Arnaud Lazarus (Ambroise Paré Clinic, Neuilly, France) awarded the manufacturers in three categories: favourite innovation, patient care improvement and practice improvement. The 2017 winners are:
Award | Company | Product |
Favourite Innovation | EBR Systems | Second generation WiSE-CRT System |
Patient Care Improvement | Spectranetics | Bridge Occlusion Balloon |
Practice Improvement | Boston Scientific | Rhythmia HDx Mapping & Navigation System |